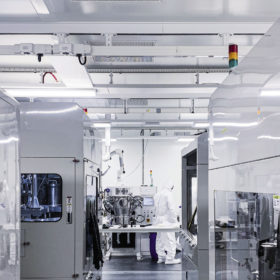
Given the number of batteries (primarily lithium-ion) that are required to decarbonize the power and mobility sectors, the industry is now preparing for a mountain of battery waste. Recycling is technically feasible, but is also subject to sensitive economics. The framework in which recycling companies can achieve the best life-cycle costs for batteries needs to be carefully enabled through policy – and it will likely include a mixture of technologies and business models, in order to allow life-cycle emissions of batteries to be brought down.
From pv magazine 08/2020
A few weeks ago, a Bloomberg interview with the chairman of Contemporary Amperex Technology, Zeng Yugun, stirred up quite a bit of media attention, causing some onlookers to rub their eyes in disbelief. Tesla’s battery supplier claims to be ready to produce batteries with a warranty for 16 years and 2 million kilometers. Hitherto, the industry standard has been around eight years and 160,000 kilometers. But nobody expects one battery pack to spend its entire life in a single electric vehicle. Already with the current warranty standard, battery packs routinely outlive the cars they are built into. Yet the million-mile battery, as it is also dubbed, isn’t going to appease the range anxiety of skeptical motorists as much as it is going to change the economics of second-life applications and the recycling of batteries.
Circular manufacturing
What is circular manufacturing, why is it important, and how is it being applied in the solar and storage industries? pv magazine’s UP initiative investigates in Q3 2020.
The economics of recycling is paramount to its execution. Engineers, entrepreneurs, and scientists are still on their quest to optimize the processes and business models around spent batteries. Technologically speaking, recycling is feasible today. Already, the lion’s share of end-of-life batteries are entering recycling processes through which most of the materials can be retrieved. The current mission is to optimize these processes to ensure the economic incentives for performing them, and find interim solutions to keep process-related energy use and emissions at the lowest possible levels.
Cathode retention
In the United States, the Department of Energy launched the ReCell collaboration between Argonne National Laboratory (ANL), which leads the initiative, National Renewable Energy Laboratory (NREL), Oak Ridge National Laboratory (ORNL), as well as Worcester Polytechnic Institute, University of California at San Diego, and Michigan Technological University, in early 2019. The project assesses different recycling methods according to their feasibility, costs and environmental performance. A life-cycle assessment tool, EverBatt, helps the researchers putting numbers to the various recycling methods and looking at how recycling economics are affected when geography and the recycling throughput change.
Linda Gaines – a chemical physicist and systems analyst with Argonne National Laboratory, and one of the ReCell project’s minds – talks about the virtues of direct recycling. Next to smelting and acid treatment, direct recycling describes the third method of battery recycling. The term captures several different processes, but they are all united by the fact that it is possible to retain the cathode morphology.
“Rather than taking it back to its elemental form, we are trying to retain the complicated crystal structure that has been developed specifically for batteries,” explains Gaines. “That cathode material might be somewhat depleted in lithium, and we are trying to put that back. One way of doing that is by taking a lithium compound and putting it in the same vessel with the cathode material and then heating it to see if you can get the reactions that are necessary to re-lithiation.”
In their analysis, the researchers found that direct recycling, albeit not yet commercially available, offers the greatest potential to generate secondary resource material flows – economically and from a life-cycle emissions perspective. That is because the cathode is the most valuable component in a battery and it also requires the largest amount of energy to produce. The average energy demand for battery manufacturing is around 170 megajoules per kWh, according to EverBatt.
For direct recycling, the cells need to be chopped and taken apart. Aluminum and copper foils can be easily separated, but then a whole range of reactions and processes is required to separate anode and cathode precursors. “And one question we have not really answered yet is exactly in which order we should be doing them in,” says Gaines. “Should you separate all the components before you upgrade them or should you upgrade and then separate?”
Direct recycling is so far incomplete when it comes to recycling lithium. When asked about the whereabouts of the depleted lithium in the battery, Gaines says that research has not yet tracked all of it. The battery can be fully discharged before direct recycling, concentrating most of the remaining lithium in one place, but some of it will be stuck between the carbon layers in the anode and in compounds on its surface. “We have one project in which we wash off that layer from the anode but haven’t fully investigated that yet. Whether you can get it back out of places where it is stuck in the layers of carbon is a good question.”
Swedish hydrometallurgy
Still, hydrometallurgy is currently commercially used and allows the retrieval of more than 90% of the battery material in purity grades that are useful for the battery industry, including lithium. Northvolt, a Sweden-based stationary battery manufacturer, has launched a joint venture with Norwegian aluminum producer Hydro for a 4 GWh recycling facility, set for operation in 2022. “I think that the main thing for the battery market going forward is that we can live up to sustainability and bring down the real footprint,” says Emma Nehrenheim, Northvolt’s chief environmental officer. “A big proportion of that is still recycling and manufacturing, but if we have a high degree of renewable energy in these processes the remaining footprint will be in the raw material supply chain.” Together with the nonprofit organization Batteriretur, Northvolt and Hydro collect used EV batteries in Norway. EV battery packs comprise 35% aluminum, which Hydro collects. Despite cobalt and lithium taking center stage in the discussion around battery recycling, aluminum deserves attention, too. Its production-related CO2 emissions from bauxite ore to ready to use metal are 12 times higher than that of iron. On top of that, it takes four to six tons of bauxite ore to produce one ton of aluminum. The difference remains as a highly toxic slurry known as red mud. In a 2010 accident in Hungary, the mud went into a river, exterminating all life. The EU, for example, attributes it to its reasoning in seeking to establish an ecodesign directive for batteries with high secondary resource content.
Another important factor for Northvolt is resilience to commodity markets. “Recycling is going to be a competitive advantage,” Nehrenheim explains. “Especially in Europe, recycling is decreasing exposure to volatile raw materials markets and it is incredibly important to become less dependent on such imports.”
After Hydro separates the aluminum black mass containing the ground-up anode and cathode, it is then shipped to a hydrometallurgical recycling facility in Sweden. By treating the black mass with various acids, valuable metals such as lithium, cobalt, nickel, and manganese can be retrieved in the form of sulfites or halide salts. By 2030 the company wants to source 50% of its raw materials from recycling processes. The demand for new batteries outpaces the supply of used ones.
Shipping and chopping
Gaines recalls an event where a battery manufacturer moved from assembling the modules with nuts and bolts to welding them together. This certainly makes recycling more complicated and likely drives costs for recyclers, but other factors likely play a more significant role.
“One of the most striking findings in EverBatt was that transportation costs of spent batteries are an important factor in getting batteries recycled,” Gaines says. “One analysis showed that it is much more economical to have several pre-treatment plants for discarded batteries than to just ship all the batteries to one big recycling plant.” Batteries are considered hazardous goods, and shipping companies charge fees on that, fearing fires and explosions.
That is a point which Northvolt also understood. The facility where Hydro extracts the aluminum is in Norway, close to where the batteries come from. The material shipped from this plant to the recycling facility in Sweden does not catch fire and is much easier to handle.
Second-life uses
As battery production and recycling requires substantial energy input and produces toxic byproducts, second-life use of end-of-life EV batteries is an essential factor in greening batteries. The market for these applications is still in its nascent stages, as calculating viable business cases for storage assets depends on debatable capacity assumptions. “It’s all about risk,” according to Graham Hall, director at CodeSmith Technology. “If I take a battery that is brand new and it breaks down, you know what is going to happen, because you have a warranty … In the second-life market, a battery might cost just one-sixth of the price, but it is a bigger risk. There is no warranty and you don’t know how many of your batteries are going to break and when. That scares companies.”
In Leeds, England, Hall and his team are trying to address the challenge of making second-life use cases economically viable. A battery’s cycle life is highly dependent on the way the battery was used. For a company to buy up stocks of spent electric vehicle batteries, it means to bet on the remaining capacity. Depending on the discharge current, full load cycles, and at what temperature batteries have been cycled, they will retain a different percentage of their original capacity.
This ambiguity has hitherto jeopardized the sensitive economics of second-life use-cases. The Battery Passport is a combination of software used by CodeSmith and hardware built into the vehicle that collects data around its use. Before entering its second-life application, the new owner can obtain an assessment of the battery’s remaining capacity. According to Hall, this is cheaper than performing individual performance tests on each battery. It is also cheaper because it allows companies to reliably calculate their risks. Currently, the Battery Passport is only used by EV producer Electra, although with interest from the industry, the concept could be expanded.
No chemistry
Incorporating second- and third-life use cases into the complete life cycle of a battery has led the recycling expert to stipulate a 30-year active life for a battery. While this is a good thing in terms of saving energy for recycling and manufacturing processes, it creates a challenge for recycling companies. The materials that are used in batteries are subject to their technological development. The widely used nickel manganese cobalt (NMC) cathode chemistry was developed to reduce cobalt proportion. Research in direct recycling thus aims to add nickel to the cathode morphology. Until there is a process that allows doing so, recyclers can only process the cathodes that they intend to reintegrate into the market.
Hydrometallurgy, meanwhile, has no such limitations. “We can take back NMC 111 batteries for example,” says Northvolt’s Nehrenheim. “We can break them down to an elementary form and make new precursors for the desired cathode composition.” The company accepts all chemistries that are high in cobalt contents for processing.
However, LFP batteries are a challenge, as the cathode contains no metals that can be used for NMC batteries, and its components are rather cheap. LFP batteries are an ongoing concern for recyclers. Not because it is technologically harder to recycle them, but simply because there is no value in doing so using hydrometallurgy. With most e-buses and Tesla’s Chinese car sales relying on LFP batteries, it could become a big issue.
Direct recycling could be the answer. “There is nothing useful essentially, except the copper from the current collectors that you will get out from smelting,” says Gaines. “You can get phosphate-ions out from hydrometallurgy. But there is really no valuable product other than the cathode itself that you can get out of an LFP battery.” And that cathode can only be retained by recycling.
Lắp đặt điện mặt trời Khải Minh Tech
https://ift.tt/2X7bF6x
0906633505
info.khaiminhtech@gmail.com
80/39 Trần Quang Diệu, Phường 14, Quận 3
Lắp đặt điện mặt trời Khải Minh Tech
https://ift.tt/2ZH4TRU
Không có nhận xét nào:
Đăng nhận xét